Circular Ribbon Flare at Microwaves
Nugget | |
---|---|
Number: | 387 |
1st Author: | Jeongwoo LEE |
2nd Author: | |
Published: | 31 August 2020 |
Next Nugget: | Deep-seated flare seismic sources |
Previous Nugget: | White-light emission and photospheric magnetic field changes in flares |
Introduction
Circular ribbon flares (CRFs) occur in a special magnetic configuration where a central parasitic magnetic field is surrounded by the major fields in the other polarity (Ref. [1]; see also previous RHESSI Nuggets 278, 381, and 382, for example). Such a configuration implies a dome-shaped separatrix with a null point on top of it; this attracts MHD modelers interested in truly three-dimensional magnetic reconnection expected around the null. Observationally EUV images have mainly been used to study such regions, and the value of microwave observations as diagnostics of CRFs has remained as an open question. We present some results from a microwave study of the CRF, SOL2014-12-17T04:51, using the Nobeyama Radioheliograph (NoRH) and argue that spatial distribution of microwave polarization and its time-dependent change may tell more about magnetic reconnection and eruption in this "fan-spine" structure.
Rapid Polarization Changes
Figure 1 shows contours of the total (yellow contours) and the polarized intensity (red/blue) at 17 GHz as measured by the NoRH. We see that all sources are left-hand circular polarized (LHCP) in the preflare phase, and on-and-after the flare, the southern source over the positive-polarity sunspot becomes right-hand circular polarized (RHCP). In theory, the emission from electrons gyrating about a positive (negative) polarity field should be RHCP (LHCP). Even without such knowledge on the exact sense of polarization, we may still wonder why the single polarization dominates above the bipolar magnetic fields, and find it more convincing when both polarizations properly appear. This change of polarization can be understood if the entire region were initially covered with coronal magnetic field with the negative polarity (i.e. the closed fan structure), which is then blown out to let the microwaves emerge in the original polarization. Evidently, a breakout-type eruption could provide the most plausible explanation for the sudden change of the microwave polarization.
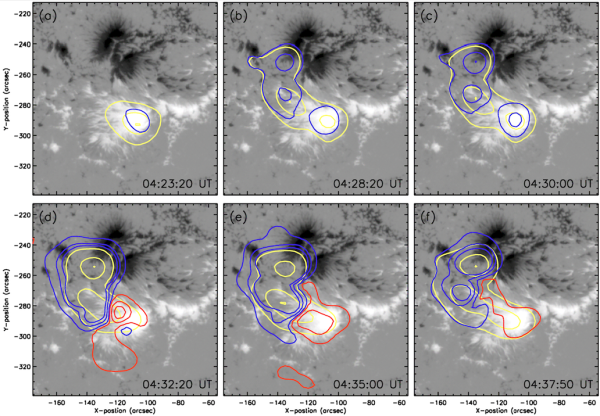
Activations and Oscillations
The overall microwave source looks like a circular disk outlined by the EUV circular ribbon with much stronger flare loop inside it. In Figure 2, we investigate local properties in the loop footpoints (A and C), the looptop (B) and the whole (D) separately (Fig. 2a). The time-distance stackplot of the SDO/AIA 131 Â intensity shows, at least, two eruption-like features (Fig. 2b), which tend to have corresponding features in the brightness temperatures of 17/34 GHz (Fig. 2c). The first rise (t1) is detected only at the 17 GHz without a counterpart at the 34 GHz and the second one (t2) impulsively rises at both 17 GHz and 34 GHz. From the known theoretical characteristics of the gyromagnetic opacity, we identify the first activation (t1) with thermal and the second as nonthermal activations (Ref. [2]). In the next two panels, we find multiple peaks (marked with arrows) in both total (Fig. 2d) and polarized intensities (Fig. 2e). They yield an impression of oscillations superimposed on the flare lightcurves, very similar to the phenomenon called quasi-periodic pulsations with a drift. The continuation of these oscillations from A to C during the eruption (t3) suggests that the oscillations on the initially closed field lines move to the erupted field lines, which serve as a conduit for the waves propagating along the spine.
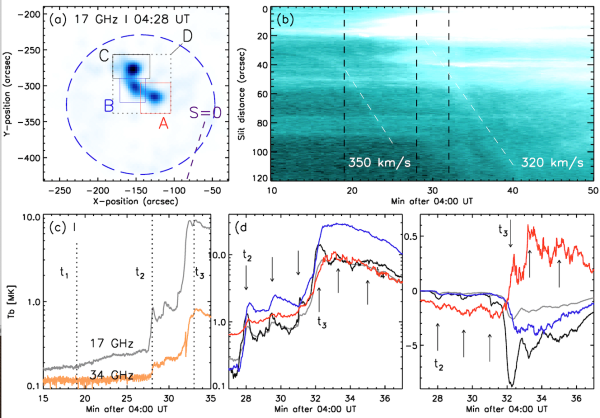
Interpretation
We interpret the above results using Figure 3, in which an ideal closed fan-spine structure with a flux rope inside evolves toward a partially open structure. (a) A perturbation occurs at t1 (Fig. 2b) to cause the null point to deform itself into a current sheet, which is not really violent but gentle so as to trigger thermal heating mostly in the inner spine (Fig. 2c). The microwaves emitted from this region must have been RHCP, but changing to LHCP while passing through the overlying fan field. (b) The next reconnection occurs at t2 between the magnetic fields inside and outside of the fan across a breakout current sheet, and the microwave source extends to the flux rope. Since this energy release is more intense, the nonthermal phase sets in (Fig. 2c) and the oscillations start everywhere (Fig. 2d,e). Again, the rays emitted from the two footpoints, initially polarized in different senses, become all LHCP while passing through the fan field. (c) In about 9 min, the breakout eruption follows to cause the flare and let the flux rope erupt. The rays from the positive field will be observed as RHCP as the overlying fan field is now open. Continuation of the oscillations through this transition implies that they are the magnetic-untwisting waves (torsional Alfven waves) propagating along the spine as guided by the erupted field lines. This scenario for explaining the microwave observations is remarkably similar to that of a recent eruption model (e.g., Ref. [3]), thus providing a strong support for recent theoretical efforts for understanding the physical processes in the fan-spine structure.
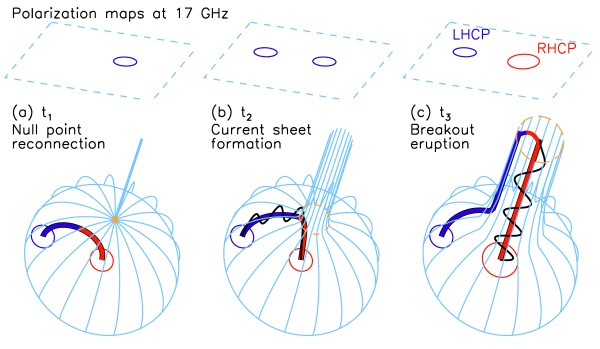
References
[1] "The Nature of Flare Ribbons in Coronal Null-Point Topology"