Proton Beam Energy Deposition as a Mechanism of Deep Photospheric Heating
Nugget | |
---|---|
Number: | 490 |
1st Author: | Samuel GRANOVSKY |
2nd Author: | Alexander KOSOVICHEV |
Published: | 17 March 2025 |
Next Nugget: | |
Previous Nugget: | New insights into the proton precipitation sites in solar flares |
Introduction
Solar flares are powerful bursts of electromagnetic radiation which occur in the solar atmosphere and release vast amounts of energy across a very wide spectrum. While they are traditionally associated with chromospheric (Hα) brightenings, they are now mainly studied via enhanced X-ray and UV emissions. Some flares exhibit significant visible continuum enhancements, and are known as white-light flares (WLFs). Despite extensive research, the mechanisms behind WLFs remain debated, particularly concerning their small, short-lived brightenings within flare kernels.
Traditional thick-target electron-beam models, which predict energy deposition in the upper chromosphere, struggle to explain the observed photospheric effects. Electron beams are known to deposit most of their energy in the chromosphere, yet photospheric line and continuum emission, and sunquakes also appear to require significant heating and hydrodynamic impacts in the lower atmosphere. Proton beams have been proposed as a possible explanation for this deeper energy penetration (Ref. [1]), but direct observational evidence remains inconclusive. Note coincidentally the inference regarding proton beams in the previous SolarNugget.
HMI Flare Observations
The SDO HMI instrument has observed a number of sunquake-producing WLFs during solar cycles 24 and 25, revealing characteristics of flare-driven photospheric heating. Its observations of the Fe I 6173 Å line emission during these flares indicate that localized brightening occurs within the umbra or along the umbra-penumbra boundary of active regions. The GOES X9.3 flare SOL2017-09-06 provided one of the clearest examples of this phenomenon, with line-core emission observed across a large portion of the umbra within the flaring active region. This major event has spawned several Nuggets already. Spectro-polarimetric analysis of five such flares reveals a number of common attributes (Refs. [2,3]). First, the peak line-core emission occurs just before or is concurrent with continuum and line-wing brightening, indicating that the emission forms above the photosphere. Second, line-core brightening lasts for less than or equal to the 90-second HMI data sampling cadence, while line-wing and continuum brightening persist for longer (3 to 20 minutes), suggesting a rapid but and localized heating process. Third, permanent changes in the solar magnetic field occur simultaneously with these emission events. Fourth, line-core brightening is confined to the sunspot umbra and penumbra regions. Finally, line-core emission takes place near the peak of hard X-ray flux, as observed by Konus-Wind, and the peak time derivative of soft X-ray flux, as recorded observed by GOES satellites.
Figure 1 shows details of the HMI observation of SOL2017-09-06.
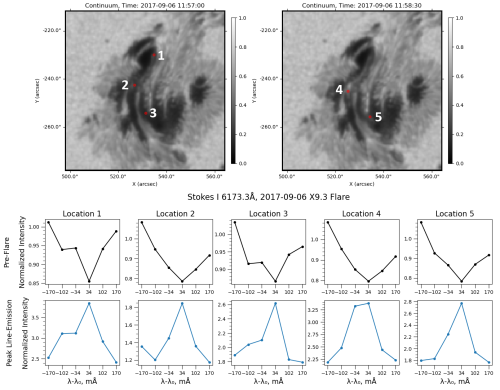
RADYN Modeling
Radiative hydrodynamic simulations using the RADYN code provide a theoretical framework for understanding the energy deposition mechanisms in solar flares. Recent RADYN-based proton beam models (Figure 2) have demonstrated significant heating in the lower chromosphere, leading to enhanced Fe I 6173 Å line emission (Ref. [4]). However, these models generally use temperature profiles characteristic of plage-like atmospheres, which may not accurately represent sunspot conditions where WLF cores are observed. By adjusting initial temperature conditions to a model more representative of sunspot umbrae, significantly stronger line-core emission is observed.
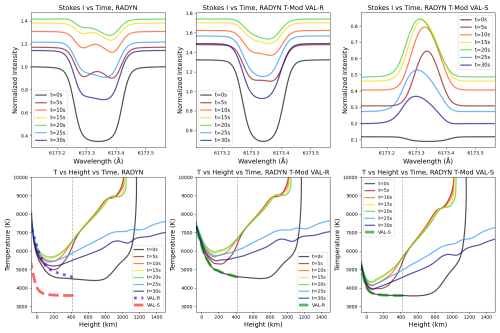
Conclusions
HMI observations of WLFs provide compelling evidence that flare energy penetrates deeper into the solar atmosphere than standard electron beam models predict. The detection of Fe I 6173 Å line-core emission, in addition to the production of sunquakes, often coinciding with sunquakes, suggests that photospheric and even subphotospheric layers are significantly impacted by flare-driven processes. Traditional thick-target models fail to account for these effects, necessitating alternative mechanisms such as proton beams.
RADYN modeling efforts indicate that electron beams alone cannot explain observed line reversals, particularly in sunspot umbrae. Instead, proton beams offer a more viable explanation, as they can deposit energy deeper in the atmosphere. However, discrepancies between proton beam energies required for Fe I emission and those that drive sunquakes suggest that additional factors may also play a role.
References
[1] "A dominant Role for Protons at the Onset of Solar Flares?"
[2] "Fe I 6173 Å Emission During White-Light Solar Flares"
[4] "Can Proton Beam Heating Flare Models Explain Sunquakes?"